The Microplastics And PFAS Connection
By Cayla Cook and Eva Steinle-Darling
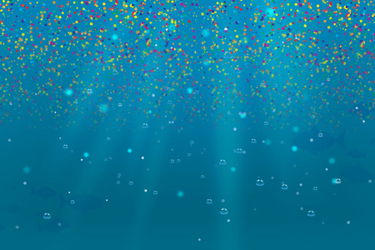
Microplastics, small plastic particles with sizes ranging from 5 millimeters to 1 nanometer with various morphologies such as microfibers, fragments, pellets (nurdles), or microbeads, have received increasing attention, including upcoming statewide monitoring in California.
Per- and Polyfluoroalkyl Substances (PFAS) are a group of unique chemically stable compounds and, as a result, have made them highly valuable across a wide range of industrial, commercial, and military uses. However, this feature concomitantly makes them recalcitrant and persistent in nature — thus coined “forever” chemicals (Lindstrom et al. 2011, Buck et al. 2011). Recent developments in toxicology, coupled with significant political pressure, have put PFAS on the fast-track for regulation in drinking water and wastewater. While co-occurrence is well-known for a variety of contaminants like triclosan and triclocarban, the connection between microplastics and PFAS has not been studied in much detail despite being linked together in multiple ways. Not only can some PFAS occur as microplastics such as polyvinyl fluoride (PVF) and polytetrafluorethylene (PTFE), it is also used as a coating on synthetic textiles and plastic components that then break down to fiber- or particle-based macro-, meso-, or microplastics. Moreover, non-PFAS microplastics can involve PFAS at certain stages in their production process, for example polyvinyl chloride (PVC).
First, it is imperative to highlight that microplastics — similarly to PFAS — are not a single type of contaminant but a suite of contaminants. There are dozens of polymer types which alone consist of 4,283 additives including the well-known endocrine disruptor detected in human blood and urine, bisphenol-A (Groh et. al 2019; Vandenberg et al. 2014; Matsumoto et. al 2003). These additives include flame retardants, plastic stabilizers, and colourants, many of which are regulated in drinking water nationwide. Recent research has indicated that “most of the chemicals present in plastics (82 percent) cannot be identified using the … [National Institute of Standards and Technology] database and, thus, remain unknown” which would increase the associated compound list into perhaps the tens of thousands (Zimmermann et al. 2019). Some of these associated polymer types and additives are also, of course, PFAS.
Perhaps most surprising is the fact that one type of PFAS, polymeric PFAS, can break down into microplastics. This polymeric PFAS group consists of fluoropolymers, side-chain fluorinated polymers, and poly- or perfluoropolyethers (Lohmann et al. 2020). PTFE and PVF may occur as secondary microplastics in the environment or as intentionally produced primary microplastics (Bergmann et al. 2017; Ebnesajjad 2013). Due to their high density, environmental PTFE and PVF microplastics are more likely to settle into sediment which makes their removal more challenging. For example, if these particles are removed in wastewater treatment, then they are likely to be found in the solids or biosolids product.
Both landfills and wastewater treatment plants are concerned about microplastics and PFAS for a variety of reasons. While the science is still developing, PFAS groundwater contamination near landfills has been found to exceed local health-based guidance values, and microplastics have been found in landfill leachate, which have contaminated nearby groundwater (Minnesota Pollution Control Agency 2021; Pinjing et al. 2019; Karuppasamy et al. 2021). Concurrently, research and regulations have been developing for PFAS in biosolids in various states due to toxicity concerns while microplastics research has begun to indicate possible land accumulation or export to the nearby environment, select agricultural crop uptake, and other impacts (Corradini et al. 2019; Crossman et al. 2020; Sun et al. 2020). While PTFE and PVF represent a small portion of microplastics discovered in the environment, fluoropolymer microplastics may further contribute to environmental, groundwater, produce, or landfill PFAS levels without the necessary source control.
Plastics, paper products, and textiles are sometimes coated in nonpolymeric PFAS coatings that can be released. This side-chain fluorinated polymer group has been found to release nonpolymeric PFAS (Washington et al. 2015). Of these compounds formed, PFAS is specifically a concern with high density polyethylene (HDPE). In March 2021, the EPA released a memo stating that “PFAS compounds can be formed then partly leach” from plastics used in pesticide packaging (EPA Releases Testing Data Showing PFAS Contamination from Flourinated Containers, 2021). Additionally, fluoride and microplastic release from heated PFAS-coated paper products has recently been documented (Ranjan, Joseph, and Goel 2021). In short, PFAS coatings on plastic may be relatively common, with microplastic release possible on some packaging including food contact substances.
Beyond packaging, fluorotelomer-based polymers (FTPs) are a PFAS category used for water repellant or stain repellant clothing coatings which are often applied to polymeric textiles such as polyamide (PA) and polyester (PES). This water-resistant clothing can shed PFAS-coated microfibers during production or subsequent consumer washing. Early research indicates that the “largest fraction of fluorine is most likely present as durable water repellant polymer chemically bound to the fiber” indicating the co-occurrence of PFAS microplastics is widespread in textile washwater (Schellenberger et al. 2019). Moreover, many of these microfibers are not removed by wastewater treatment processes in either textile treatment facilities or municipal wastewater treatment plants (Ranjan, Joseph, and Goel 2021; Le Bihanic et al. 2020). An estimated ~0.7 tons of FTPs per year are emitted by outdoor rain jackets alone (Schellenberger et al. 2019).
In addition to polymeric PFAS and PFAS-coated plastics and textiles, PFAS can also adsorb onto microplastics in the environment then possibly desorb in aquatic species but not the human gut (Wang, Shih, and Li 2015; Islam et al. 2021; Bakir, Rowland, and Thompson 2014). Adsorption or association with more than 78 percent of the Environmental Protection Agency’s priority pollutants further links macro-, meso-, and microplastics not just to PFAS, but other environmental contaminants such as polychlorinated biphenyls (PCBs), dichlorodiphenyltrichloroethane (DDT), and pharmaceuticals (Rochman et al. 2013). Dependent upon the pH, salinity, and other factors, microplastics can adsorb to these hydrophobic contaminants and then desorb in more preferential conditions.
To bring this full circle, microplastics and PFAS are often found together in the environment and recent research has indicated that microplastics may increase PFAS toxicity (Pramanik et al. 2020; Le Bihanic et al. 2020; Sobhani et al. 2021). The co-occurrence of these two contaminants may come as a surprise to the public, utilities, or industries as each has historically been treated as an isolated issue; however, this is not always the case. Simultaneous microplastics and PFAS monitoring by utilities and industries can begin to shed light and increase knowledge on this co-occurrence. Moreover, source control of PFAS-coated textiles which shed microfibers could have a concurrent, positive impact on environmental and potable water quality. Increasing our understanding on their co-occurrence and considering concurrent mitigation strategies, including source control and treatment technologies, will lead to long-term sustainable solutions.
Cayla Cook is a Microplastics Lead and Staff Engineer with Carollo Engineers. She has a BSE in Civil and Environmental Engineering from Mississippi State University and an M.S. in Environmental Engineering from Arizona State University, where she studied smart polymers, biopolymer nanotechnology, microplastic analytical methods, and environmental and human health impacts of plastic and plastic-associated additives. Cayla spearheads all micro- and nanoplastic-related efforts at Carollo for water and wastewater. This includes investigating mitigation strategies including treatment technologies, implementing monitoring initiatives, and building a database to advance understanding of contamination.
Eva Steinle-Darling, PE, is a Vice President and Chief Technologist with Carollo Engineers. She has a BSE in Chemical Engineering from Princeton University and an M.S. and Ph.D. in Environmental Engineering from Stanford University, where she studied the removal of PFAS using nanofiltration membranes. Her work with Carollo focuses on advanced water treatment and reuse, including research, planning, feasibility studies, and design. Eva is leading Carollo's efforts to address clients' needs in responding to PFAS contamination in drinking water, wastewater, and recycled water. She also is working on several of Carollo’s Water Research Foundation projects in which she is looking at, among many other things, the removal of PFAS through the advanced treatment train.
References
Bakir, Adil, Steven J. Rowland, and Richard C. Thompson. 2014. "Enhanced desorption of persistent organic pollutants from microplastics under simulated physiological conditions." Environmental pollution 185 (February): 16–23.
Bergmann, Melanie, Vanessa Wirzberger, Thomas Krumpen, Claudia Lorenz, Sebastian Primpke, Mine B. Tekman, and Gunnar Gerdts. 2017. "High quantities of microplastic in Arctic deep-sea sediments from the HAUSGARTEN observatory." Environmental Science & Technology 51 (19): 11000–11010.
Buck, Robert C., Peter M. Murphy, and Martial Pabon. 2012. “Chemistry, Properties, and Uses of Commercial Fluorinated Surfactants.” In Polyfuorinated Chemicals and Transformation Products, 1–24. Berlin: Springer-Verlag. https://doi.org/10.1007/978-3-642-21872-9.
Corradini, Fabio, Pablo Meza, Raúl Eguiluz, Francisco Casado, Esperanza Huerta-Lwanga, and Violette Geissen. 2019. "Evidence of microplastic accumulation in agricultural soils from sewage sludge disposal." Science of the Total Environment 671: 411–420.
Crossman, Jill, Rachel R. Hurley, Martyn Futter, and Luca Nizzetto. 2020. "Transfer and transport of microplastics from biosolids to agricultural soils and the wider environment." Science of the Total Environment 724: 138334.
Ebnesajjad, Sina. 2013. Introduction to Fluoropolymers: Materials, Technology and Applications. Watham, MA: William Andrews.
EPA Releases Testing Data Showing PFAS Contamination from Flourinated Containers. Environmental Protection Agency, 05 March 2021, https://www.epa.gov/newsreleases/epa-releases-testing-data-showing-pfas-contamination-fluorinated-containers. Press Release.
Groh, Ksenia J., et al. 2019. "Overview of known plastic packaging-associated chemicals and their hazards." Science of the Total Environment 651 (Part 2): 3253–3268.
He, Pinjing, Liyao Chen, Liming Shao, Hua Zhang, and Fan Lü. 2019. "Municipal solid waste (MSW) landfill: A source of microplastics?-Evidence of microplastics in landfill leachate." Water Research 159: 38–45.
Islam, Naimul, Tainá Garcia da Fonseca, Juliano Vilke, Joanna M. Gonçalves, Paulo Pedro, Steffen Keiter, Sara C. Cunha, José O. Fernandes, and M.J. Bebianno. 2021. "Perfluorooctane sulfonic acid (PFOS) adsorbed to polyethylene microplastics: Accumulation and ecotoxicological effects in the clam Scrobicularia plana." Marine Environmental Research 164 : 105249.
Karuppasamy, Manikanda Bharath, Usha Natesan, Vaikunth R., Praveen Kumar R, Ruthra R, and ‪Seshachalam Srinivasalu. 2021. "Spatial distribution of microplastic concentration around landfill sites and its potential risk on groundwater." Chemosphere: 130263.
Le Bihanic, Florane, Christelle Clérandeau, Bettie Cormier, Jean-Claude Crebassa, Steffen H. Keiter, Ricardo Beiras, Bénédicte Morin, Marie-Laure Bégout, Xavier Cousin, and Jérôme Cachot. 2020. "Organic contaminants sorbed to microplastics affect marine medaka fish early life stages development." Marine Pollution Bulletin 154: 111059.
Lindstrom, Andrew B., Mark J. Strynar, Amy D. Delinsky, Shoji F. Nakayama, Larry McMillan, E. Laurence Libelo, Michael Neill, and Lee Thomas. 2011. “Application of WWTP Biosolids and Resulting Perfuorinated Compound Contamination of Surface and Well Water in Decatur, Alabama, USA.” Environmental Science & Technology 45 (19): 8015–21. https://doi. org/10.1021/es1039425.
Lohmann, Rainer, et al. 2020. "Are fluoropolymers really of low concern for human and environmental health and separate from other PFAS?." Environmental Science & Technology 54 (20): 12820–12828.
Matsumoto, Akiko, Naoki Kunugita, Kyoko Kitagawa, Toyohi Isse, Tsunehiro Oyama, Gary L Foureman, Masatoshi Morita, and Toshihiro Kawamoto. 2013. "Bisphenol A levels in human urine." Environmental Health Perspectives 111, no. 1 (January): 101-104.
Minnesota Pollution Control Agency. 2021. “Nearly 60 closed landfills in 41 counties have PFAS contamination in groundwater that exceeds the state's health value. News release, March 18, 2021. https://www.pca.state.mn.us/news/nearly-60-closed-landfills-pfas-contamination-groundwater-exceeds-state-health-values.
Pramanik, Biplob Kumar, Rajeev Roychand, Sirajum Monira, Muhammed Bhuiyan, and Veeriah Jegatheesan. 2020. "Fate of road-dust associated microplastics and per-and polyfluorinated substances in stormwater." Process Safety and Environmental Protection 144 (December): 236–241.
Ranjan, Ved Prakash, Anuja Joseph, and Sudha Goel. 2021. "Microplastics and other harmful substances released from disposable paper cups into hot water." Journal of Hazardous Materials 404: 124118.
Rochman, Chelsea M., Mark Anthony Browne, Benjamin S. Halpern, Brian T. Hentschel, Eunha Hoh, Hrissi K. Karapanagioti, Lorena M. Rios-Mendoza, Hideshige Takada, Swee The, and Richard C. Thompson. 2013. “Classify plastic waste as hazardous.” Nature 494 (7436): 169–171.
Schellenberger, Steffen, et al. 2019. "Release of side-chain fluorinated polymer-containing microplastic fibers from functional textiles during washing and first estimates of perfluoroalkyl acid emissions." Environmental Science & Technology 53.24: 14329–14338.
Sobhani, Zahra, Cheng Fang, Ravi Naidu, and Mallavarapu Megharaj. 2021. "Microplastics as a vector of toxic chemicals in soil: Enhanced uptake of perfluorooctane sulfonate and perfluorooctanoic acid by earthworms through sorption and reproductive toxicity." Environmental Technology & Innovation 22 (May): 101476.
Sun, Xiao-Dong, et al. 2020. "Differentially charged nanoplastics demonstrate distinct accumulation in Arabidopsis thaliana." Nature Nanotechnology 15.9: 755–760.
Vandenberg, Laura N., et al. 2014. "A round robin approach to the analysis of bisphenol A (BPA) in human blood samples." Environmental Health 13 (1): 1-20.
Wang, Fei, Kai Min Shih, and Xiao Yan Li. 2015. "The partition behavior of perfluorooctanesulfonate (PFOS) and perfluorooctanesulfonamide (FOSA) on microplastics." Chemosphere 119: 841–847.
Washington, John W., Thomas M. Jenkins, Keegan Rankin, and Jonathan E. Naile. 2015. "Decades-scale degradation of commercial, side-chain, fluorotelomer-based polymers in soils and water." Environmental Science & Technology 49.2: 915–923.
Zimmermann, Lisa, Georg Dierkes, Thomas A. Ternes, Carolin Völker, and Martin Wagner. 2019. "Benchmarking the in vitro toxicity and chemical composition of plastic consumer products." Environmental Science & Technology 53 (19): 11467–11477.