Radionuclides in Drinking Water: a Problem That Must Be Dealt With
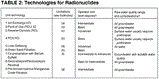
The potential for radioactive substances to present problems exists throughout the United States as releases from medical facilities or nuclear power plants may wind up in drinking water. Because of their potential health effects and widespread occurrence, natural radionuclides—including radon, radium, and uranium—cause much concern.
Contents
Where do radionuclides occur, and what are the public health risks?
What water treatment process can remove radionuclides?
Sources for more information
Radionuclide contamination of drinking water is a significant, emerging issue. Until recently, decades manmade radioactivity in drinking water has not been a major problem. Natural sources have been the primary cause of this type of contamination. However, the potential for these radioactive substances to present problems exists throughout the country as releases from medical facilities or nuclear power plants may wind up in drinking water. Because of their potential health effects and widespread occurrence, natural radionuclides—including radon, radium, and uranium—cause much concern.
Where do radionuclides occur, and what are the public health risks? (top)
Radionuclides occur naturally as trace elements in rocks and soils as a consequence of the "radioactive decay" of uranium-238 (U-238) and thorium-232 (Th-232). This decay occurs because radioactive atoms have an excess of energy. When these atoms release or transfer their extra energy, the phenomenon is known as decay. The energy they release is called ionizing radiation, which may be in the form of alpha particles, beta particles, or gamma rays. This energy is transmitted through space or some other medium in waves (e.g., x-rays or gamma rays) or particles (e.g., electrons or neutrons) and is capable of either directly or indirectly removing electrons from atoms, thereby creating ions, which are electrically charged atoms.
Radon-222, radium-226, radium-228, uranium-238, and uranium-234 are ions of the U-238 and Th-232 decay series. They are the most common radionuclides found in groundwater. Other naturally occurring radionuclides tend to be environmentally immobile or have short half-lives, meaning they are far less likely to be found in significant amounts in groundwater.
When ionizing radiation strikes a living organism's cells, it may injure these cells. If radiation affects a significant number of them, the organism may eventually develop cancer. At extremely high doses it may even die.
Radon is a naturally occurring radioactive gas that emits ionizing radiation. National and international scientific organizations have concluded that radon causes lung cancer in humans. Ingesting drinking water that contains radon also presents a risk of internal organ cancers, primarily stomach cancer. The U.S. Environmental Protection Agency (EPA) and the U.S. Surgeon General recommend testing indoor air for radon in all homes and apartments located below the third floor. Persons who smoke and whose homes exhibit high indoor radon levels are at especially high risk level and they could develop lung cancer.
Tap water only emits approximately 1% to 2% of the radon found in indoor air. However, breathing radon from this source increases the risk of lung cancer over the course of a lifetime.
Radium-226 and radium-228 are natural groundwater contaminants that usually occur in trace quantities. At high exposure levels, radium-226 and radium-228 can cause bone cancer in humans and are believed to cause stomach, lung, and other cancers as well.
Uranium is a naturally occurring radioactive contaminant found in both groundwater and surface water. At high exposure levels, uranium is believed to cause bone cancer and other cancers in humans. EPA also believes that uranium can be toxic to the kidneys.
Gross Alpha emitters occur naturally as radioactive contaminants, but several come from manmade sources. They may occur in either groundwater or surface water. At high exposure levels, alpha emitters are believed to cause cancer in humans.
Beta and photon emitters are primarily manmade radioactive contaminants associated with operating nuclear power plants, facilities that use radioactive material for research or manufacturing, or facilities that dispose of radioactive material. Some beta emitters occur naturally. Beta and photon emitters primarily occur in surface water. At high exposure levels, beta and photon emitters are believed to cause cancer in humans.
The EPA rules covering the various aspects of the radionuclide issue are shown in the table. Acronyms used in the table are explained below in the section reviewing treatment options for removal of radionuclides from drinking water.
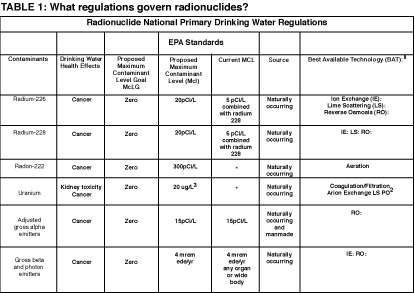
- Except as noted, BAT for the purpose of issuing variances is the same as BAT for compliance.
- Coagulation/Filtration and Lime Softening are not BAT for small systems (those with fewer than 500 connections) for the purpose of granting variances.
- 20 µg/L is based on kidney toxicity. 20 µg/L is the equivalent of 30 pCi/L.
- PicoCuries per liter (pCi/L) is an activity measurement of radioactive decay (1 pCi/L = 22 disintigrations per min); micrograms per liter (µg/L) is a mass measurement; mrem is measurement of effective radiation dose to organs.
Note: EPA recognizes that most radionuclides emit more than one type of radiation as they decay. The lists of compounds labeled "alpha" or "beta" emitters identify the predominant decay mode.
Note: In this document the unit mrem ede/yr refers to the dose ingested over 50 years at the rate of 2 liters of drinking water per day.
Source: U.S. Environmental Protection Agency
What water treatment process can remove radionuclides? (top)
Whether or not a particular treatment technology effectively removes radionuclides from drinking water depends on the contaminant's chemical and physical characteristics as well as the water system's characteristics (e.g., the source water quality and the water system size). Other considerations include cost, service life and co-treatment compatibility.
The following treatment process systems have been evaluated for their ability to remove radionuclides from water:
- ion exchange (IE)
- point-of-use IE
- point-of-entry IE
- reverse osmosis (RO)
- point-of-use RO; point-of-entry RO
- lime softening (LS)
- greensand filtration
- co-precipitation with barium sulfate
- selective sorbents
- electrodialysis/electrodialysis reversal (ED/EDR)
- preformed hydrous manganese oxides (HMOs)
Ion Exchange: Small systems may readily use IE treatment, which removes approximately 90% of radionuclides. The effluent must be regularly monitored and the IE resin must be frequently regenerated to ensure that breakthrough does not occur. Ion exchange units may be controlled automatically, requiring less of the operator's time. However, it is necessary to employ a skilled operator to determine when regeneration is needed and to trouble-shoot. Also, disposal of concentrated radionuclides can be expensive.
The ion exchange process generates wastes that include rinse and backwash water, and the IE resin. The rinse and backwash liquid waste includes brine, radium, and any other contaminants that the process removes.
Cation Exchange: A cation is a positively charged ion. Cation exchange resins exchange like-charged ions equally with protons—sodium ions (Na+), or in sodium-restriction cases, potassium ions (K+)—to remove undesirable cations from water. Cation exchange is often used to remove calcium and magnesium cations, and to treat hard water.
The quantity of waste in the rinse and backwash water that the cation exchange process typically generates ranges between 2% and 10% of the treated water.
Lime Softening: This very common process used treat hard water also can be applied to remove radium from drinking water with 80% to 95% efficiency. Also, adding lime or lime-soda ash to water increases the pH of the water and induces calcium carbonate and magnesium hydroxide precipitation.
Lime softening generates wastes that include lime sludge, filter backwash liquid and sludge, and the supernatant liquid from the sludge.
Reverse Osmosis: This system can remove many inorganic contaminants very effectively, including heavy metals and radionuclides such as radium and uranium. RO can remove 87% to 98% of radium from drinking water. Similar elimination can be achieved for alpha particle activity and total beta and photon emitter activity.
When an RO system is being used to remove radionuclides, its performance depends on a number of factors. These include pH, turbidity, iron/manganese concentration in the raw water, and the type of membrane employed. Pretreatment of the water fed to an RO system is required and the process chosen to accomplish that depends on the quality and quantity of the source water. Existing treatment plants may already provide much of the required pretreatment—for example, coagulation/filtration of highly turbid surface water or iron removal for a well water source. Reverse osmosis can be a cost-effective solution for small systems.
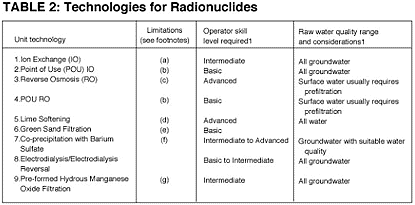
- National Research Concil (NRC). "Safe Water form Every Tap: Improving Water Service to Small Coummunities." National Academy Press. Washington, DC. 1997.
Limitations Footnotes
a. The regeneration solution contains high concentrations of the contaminant ions. Disposal options should be carefully considered before choosing this technology.
b. When POU devices are used for compliance, programs for long-term operaion, maintenance, and monitoing must be provided by the water utility to ensure proper performance.
c. Reject water disposal options should be carefully considered before choosing this technology.
d. The combination of variable source water quality and the complexity of the chemistry involved in lime softening may make this technology too complex for small surface water systems.
e. Removal efficiencies can vary depending on water quality.
f. This technology may be very limited in application to small systems. Since the process requires static mixing, detention basins, and filtration; it is most applicable to systems with sufficiently high sulfate levels that already have a suitable filtration treatment train in place.
g. This technology is most applicable to small systems that already have filtration in place.
Source: Environmental Protection Agency, 1998.
The following processes were not listed as best available technology (BAT) systems for radionuclide removal by the EPA in the 1991 proposal.
Greensand Filtration for Radium Removal: A greensand filtration system utilizes a conventional filter box with manganese greensand replacing traditional filtration media. Studies have demonstrated that this process can remove up to 56% of radium.
The wastes generated by this method include sludge and supernatant liquid from the filter backwash, and eventually the greensand medium must be disposed of.
Preformed Hydrous Manganese Oxide Filtration: The cost for HMO filtration can be quite low if a filter system already is in place. The process is similar to oxidation/filtration in its complexity and the operator skill it requires. Proper dosages must be determined, and if water quality varies, the dosage must be re-calibrated. Once the proper dose is determined, dosing is relatively easy. HMO filtration requires simple equipment and is fairly inexpensive.
Filters must be backwashed, which may require intermediate operator skill. Radium-containing wastes include HMO sludge, filter backwash, and sludge supernatant.
Co-precipitation of Radium with Barium Sulfate: Adding a soluble barium salt—such as barium chloride—to radium- and sulfate-contaminated water causes co-precipitation of a highly insoluble radium-containing barium sulfate sludge. This process has primarily been used for wastewater treatment. Mine wastewater treatment data indicates that this process removes up to 95%S of radium.
The process generates wastes that include the sludge containing the barium sulfate precipitate, filter backwash, and sludge supernatant.
Other Technologies Available: Some other systems that may have the potential to become useful processes for removing radionuclides from drinking water also are available. However, these have not been adequately tested for that purpose, or they have been used only in industrial or experimental situations. Examples of processes that can remove radium include selective sorbents (e.g., acrylic fibers or resins impregnated with manganese dioxide) and non-sodium cation exchangers (e.g., hydrogen ions and calcium ions).
Sources for more information (top)
- American Water Works Association & American Society of Civil Engineers. 1998. Water Treatment Plant Design; 3rd ed. The McGraw-Hill Companies, Inc.
- U. S. Environmental Protection Agency. September 1998. Small System Compliance Technology List for the Non-Microbial Contaminants Regulated Before 1996. EPA 815-R-98-002.
- U.S. Environmental Protection Agency. August 1998. Federal Register/Notices. Vol. 63, No. 151.
- U. S. Environmental Protection Agency. October 1999. Office of Groundwater and Drinking Water. Proposed Radon in Drinking Water Rule: Technical Fact Sheet EPA 815-F-99-006. www.epa.gov/safewater/radon/fact.html
- U. S. Environmental Protection Agency. June 1991. Office of Ground Water and Drinking Water. Radionuclides in Drinking Water - Fact Sheet EPA 570/9-91-700.
- U. S. Environmental Protection Agency. July 1991. "National Primary Drinking Water Regulations; Radionuclides; Proposed Rule." Federal Register, Vol. 56, No. 138.
- U. S. Environmental Protection Agency. March 1997. Federal Register, Vol. 62, No. 43.
Edited by Editor Emeritus, Ian Lisk, this article is based on a Tech Brief prepared by the National Drinking Water Clearinghouse located on the campus of West Virginia University at Morgantown, WV. For more information call 800-624-8301 or 304-293-4191. The NDWC Website address is http://www.ndwc.wvu.edu.