PFAS In Wastewater: Promising Management And Treatment Strategies
By Jay R. Surti, PE, with Charles Winslow, PE, and Beth Landale, PE
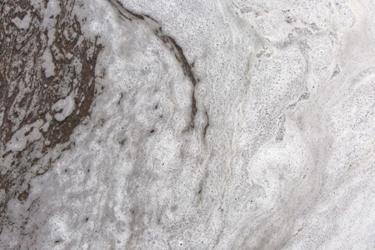
A review of emerging PFAS treatment technologies, including case studies, to help operators evaluate options for mitigation
The presence of “forever chemicals” in wastewater is a significant impediment for water and resource recovery facilities (WRRFs) to recover resources (water, energy, nutrients) and serve as anchors for the circular economy and a decarbonized future. These chemicals, formally named per-and polyfluoroalkyl substances (PFAS), enter WRRFs directly through sewage and industrial effluent, including landfill leachate and septage. Sewage and industrial effluent often contain PFAS because of widespread use of PFAS-containing materials by industry and consumers. PFAS therefore end up in both the liquid and solids streams of treated wastewater, and from there to landfills, groundwater, receiving waterbodies, and farmland through biosolids land application programs.
PFAS Health Effects And Characteristics
Since the first manufactured chemical in the PFAS family was invented in 1947, these compounds have proliferated, and there are now more than 5,000 species. The widespread use over the past several decades has led to the omnipresence of PFAS in the environment through manufacturing waste streams and disposal of PFAS-containing materials. PFAS have gained national attention because they are harmful to human health, they persist in the environment, and they are challenging to treat. While studies are ongoing and there is still much to learn, peer-reviewed studies indicate that at certain exposure levels, some chemicals in the PFAS family may lead to reproductive effects in pregnant women, developmental delays in children, increased cancer risks, lower immune response, hormone interference, increased cholesterol levels, and increased risk of obesity.
An understanding of PFAS chemistry is essential for understanding the refractory (resistant) and persistent nature of PFAS chemicals and why they are difficult to treat. Terminal perfluoroalkyl acids (PFAAs) — those such as PFOA and PFOS, which are the end degradation products of larger PFAS molecules — are extremely stable both thermally and chemically, and they resist degradation and oxidation. Their thermal stability is primarily attributable to the strength of the carbon-fluorine (C-F) bond. There is a growing interest in commercially viable thermal treatment technologies such as incineration, gasification, and pyrolysis for PFAS destruction because of the high energy required to break the C-F bond. Other technologies gaining attention for PFAS destruction include advanced oxidation processes, photocatalysis, UV irradiation, electron beam, and plasma-based treatment. However, these technologies are still in the early research and development phase. As PFAS contamination limits resource recovery and restrictive regulations increase, solutions to the PFAS challenge become ever more urgently needed.
Evaluation of full-scale WRRFs has indicated that conventional primary (sedimentation and clarification) and secondary (biological) treatment processes can result in changes in PFAS concentrations and classes such as increased PFAA concentrations in effluent compared with concentrations in influent, presumably from degradation of precursor PFAS. Limited research has been performed to understand the fate of PFAS during commercially viable solids stabilization processes that are typically employed to produce safe and high-quality biosolids by reducing pathogens and the potential to attract vectors.
PFAS Management And Treatment Strategies
Technologies and solutions are being implemented for reducing WRRF risks by treating PFAS at the source, or capturing or treating them in wastewater and biosolids matrices. PFAS management and treatment strategies can be broadly classified as follows:
- Source control to reduce PFAS load to WRRFs (discussed below)
- Separation and likely destruction of PFAS during wastewater treatment (illustrated with two case studies).
Managing PFAS Before They Enter WRRFs
WRRFs are passive receivers of PFAS chemicals. Source control is the most efficient way to reduce PFAS loads in wastewater influent. Existing industrial pretreatment programs (IPPs) for managing chemicals in wastewater from industrial facilities may be useful for PFAS source control. However, although source control can reduce PFAS concerns for utilities, it may increase operational challenges and costs for upstream industrial contributors.
Several major WRRFs significantly reduced PFOS discharges using point source treatment under Michigan’s PFAS IPP initiative. Seven used granular activated carbon (GAC), with a few adding treatments such as columns with resins and changing the water supply. Table 1 compares effluent PFOS levels for several of these facilities after they implemented source control, with a goal of reaching Michigan’s water quality standard (WQS) of 12 ppt for non-potable surface water sources. Several utilities reduced levels below this WQS.
Treating PFAS: Containing Groundwater And Biosolids
Case Study: Biosolids Pyrolysis — Ephrata, PA
Introduction
With concerns about the presence of PFAS in biosolids and their potential impact on biosolids beneficial use programs across North America, there is an urgent need to develop mitigation and treatment solutions for PFAS in biosolids. The Ephrata Borough Authority owns and operates two municipal wastewater treatment plants (WWTPs) in Lancaster County, Pennsylvania. The design-rated capacity of WWTP #1 is 3.8 MGD. With equipment at WWTP #1 aging, rising costs of landfill disposal, and the likelihood of new PFAS regulations in the future, the Authority sought to determine an optimal solution to address biosolids operational and disposal challenges, reduce reliance on third-party contractors, and enhance resilience and sustainability.
The Authority decided to implement biosolids pyrolysis because lifecycle costs would be lower and the construction sequence and schedule would be more favorable than upgrading the existing anaerobic digestion process. In addition, existing digesters could be used for sludge storage and to reduce high-strength nutrient loads being returned to the liquid treatment process. The BioforceTech® biodrying and pyrolysis process was selected for the project. Preliminary analytical testing conducted by the technology provider has demonstrated non-detect levels of 38 PFAS compounds in biochar produced through pyrolysis treatment of anaerobically digested biosolids. GHD provided the design, permitting, and construction-phase services for the new pyrolysis system. Figure 1 is a process flow diagram of the upgraded WWTP #1 solids treatment process after implementation of the biosolids pyrolysis process.
Table 1: Michigan IPP Initiative To Treat PFAS At The Source
Source: Michigan IPP Initiative, Identified Industrial Sources of PFOS to Municipal Wastewater Treatment Plants, Aug. 2020
a. Greater than Michigan’s Water Quality Standard of 12 ppt.
b. AFFF = aqueous film forming foam
Biosolids Pyrolysis And PFAS
Recently there has been renewed interest in implementing thermal oxidation processes to allow the beneficial use of wastewater biosolids while achieving significant weight and volume reduction. Pyrolysis is a nonbiological thermochemical process in which volatile organic compounds and some carbonaceous materials in the feedstock are converted into a combustible syngas (also known as synthetic gas, synthesis gas, or producer gas) in a high-temperature, oxygen-free environment, leaving behind a small amount of biochar. Char consists of both inert materials (ash) and carbonaceous materials not volatilized into a gas phase.
Pyrolysis — literally meaning “to break with fire” — occurs after the majority of the water has been evaporated from biosolids. During pyrolysis, the combination of high temperatures (600°F to 1,200°F) and an oxygen-starved environment causes volatilization of organics. These include low molecular weight gases as well as tars and oils. Figure 2 is a process flow diagram of a close-coupled biosolids pyrolysis system utilizing a municipal wastewater solids feedstock.
Figure 1. New biodrying + pyrolysis system at Ephrata Borough Authority’s WWTP #1
As of this writing, there are currently no U.S. EPA-mandated regulations for PFAS compounds in municipal wastewater effluent discharges or wastewater biosolids. However, concern about the occurrences of PFAS compounds in drinking water and groundwater could likely lead to future regulation and monitoring of PFAS in wastewater effluent discharges and wastewater biosolids. Thermal technologies such as pyrolysis have been shown to be capable of producing biochar that does not contain detectable levels of PFAS. Active research is underway to assess the efficacy and pathway of PFAS destruction in a close-coupled biosolids pyrolysis system.
Case Study: PFAS Treatability Studies For A Site In Michigan
Introduction
At a former Michigan industrial site, PFOA and PFOS were found in groundwater, although only PFOS was present above the Michigan surface water WQS. In 2019, efforts began to develop a cost-effective plan to remove them and other contaminants (iron, phosphorus, and oil and grease) to meet standards for discharge under a National Pollutant Discharge Elimination System permit.
GHD developed a conceptual design for a treatment sequence and conducted bench-scale studies on the efficacy of (1) pretreatment, (2) two adsorption technologies shown to be effective, and (3) an emerging destructive technology. The short-term goal was to find the most cost-effective conventional treatment for the PFAS compounds currently regulated, PFOA and PFOS. Longer term, the goal was to begin investigating emerging technologies that could destroy the PFAS captured in treatment media, so the financial costs of disposal and the environmental costs of continued presence could be avoided. In GHD’s fully equipped treatability laboratory in Niagara Falls, New York, scientists and engineers tested treatments for PFOA and PFOS, as well as 10 other PFAS compounds on Michigan’s Environment, Great Lakes and Energy (EGLE) analyte list but not yet regulated (12 additional PFAS compounds are on the list but were not found in the site’s groundwater).
Figure 2. Process flow for a close-coupled biosolids pyrolysis system
Conceptual Design Of The Treatment Train
GHD designed a treatment sequence that included pretreatment with ferric chloride to coagulate the iron and other contaminants in the groundwater, which would otherwise reduce the lifecycle of adsorptive media, and to precondition the groundwater for PFAS removal through adsorption (GAC or ion exchange [IX]). The coagulated solids would be removed through settling. Bag filters would then remove non-settleable and colloidal particles, and the pretreated stream would be conveyed through adsorption vessels for removal of PFAS (Figure 3).
Figure 3: PFAS-contaminated groundwater treatment steps
Treatability Studies
Pretreatment, GAC, and IX were tested in the lab, as well as several other treatment options not discussed herein.
Pretreatment: Consider the entire unique stream.
No two contaminated liquid streams are the same. Whether planning for groundwater or wastewater treatment, the effect of all contaminants on the treatment process and efficiency must be considered. The results of the pretreatment study highlighted the need to develop appropriate pretreatment to remove co-contaminants that could impede or reduce the efficiency of PFAS treatment. The effects of other contaminants on the lifecycle of the treatment media, and thereby the cost-effectiveness of the treatment plan, are also important to consider. The bench-scale studies identified effective processes and ferric chloride (coagulant) concentrations needed to remove iron and other contaminants to pretreat this groundwater stream.
Adsorption using GAC: Proven approach #1
Adsorption kinetics differ, depending on the specific PFAS compound and its affinity for carbon adsorption. GAC from various manufacturers can also work better for some streams than for others. GAC from two manufacturers was tested at bench scale for its ability to adsorb the PFAS compounds from pretreated and non-pretreated influent. Media from both manufacturers were effective in removing PFOS (the only regulated PFAS above WQS) to below the WQS. In addition, both types removed phosphorus to below its WQS. And in both, shorter-chain PFAS broke through the adsorption columns first. Although the primary focus of the treatability studies was to assess the removal efficiency of PFOA and PFOS, it was concluded that the affinity of different PFAS compounds for adsorption can vary. The design of the adsorption system would need to be flexible such that it could be modified to achieve removal of other PFAS compounds if subjected to regulations in the future.
Adsorption using IX: Proven approach #2
PFOS did not break through at all on the IX column, and the IX resin achieved higher throughput per unit mass of media than did the GAC. As with the GAC, shorter chains broke through more quickly than longer chains in the IX column.
GAC and IX compared
Both GAC and IX removed PFOS below the WQS, but both result in residuals (adsorption media) containing PFAS that must be destroyed or managed carefully. A lifecycle evaluation is required to determine the lifecycle costs of GAC and IX adsorption systems to allow comparison. GAC was chosen as the adsorption medium for the temporary treatment system because of its easy availability and the client’s prior experience. Lessons learned during the temporary treatment system include the high fouling potential of GAC media because of the presence of iron.
Conclusion
The information discussed herein will help utility owners, operators, and engineers increase understanding of the growing concerns about PFAS for the wastewater industry, the evolving regulatory environment, and promising management and treatment strategies to address PFAS-related challenges. PFAS risk management and treatment technologies are evolving in parallel to proposed federal and state regulatory guidance criteria. Each technology has pros and cons related to pretreatment requirements, individual PFAS removal rates, and ease of implementation at the full-scale level.
Destructive PFAS treatment technologies to mitigate long-term risks and liabilities for end users are emerging as practical solutions and reaching commercial scale. The case studies discussed herein suggest that destructive technologies exist but require careful evaluation to fully understand byproducts formed during the process, transformation pathways, and associated capital and lifecycle costs.
In parallel with these evaluations, we recommend utilities act now to evaluate operational risks and develop mitigation plans to address the regulatory uncertainty related to PFAS. This could include actions such as:
- Characterization and fate and transport of PFAS throughout operations, including in water, wastewater, biosolids, and spent media.
- Evaluation of existing treatment systems and their ability to remove or treat PFAS.
- Treatability trials to develop PFAS removal (separation) and destruction solutions.
- Adaptive planning in light of regulatory uncertainty.
- Evaluation of alternative approaches for managing biosolids produced during wastewater treatment, especially if they will be applied to land.
About The Author
Jay Surti, PE (jay.surti@ghd.com), is the East Region Biosolids Leader with GHD. He is a Professional Engineer with more than 20 years of experience serving the water and wastewater industry, providing innovative solutions to renew aging infrastructure, enhance sustainability and resiliency, and address contaminants of emerging concern such as PFAS. Jay is a licensed Professional Engineer, and has a Bachelor of Engineering and a Master of Engineering degree in Environmental Engineering from Stevens Institute of Technology, New Jersey.