Novel Oxidant Lowers Natural Organic Matter Without Creating Disinfection Byproducts
By Emma Flanagan
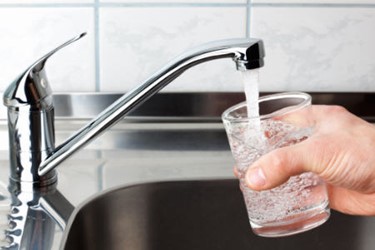
With twice the oxidation potential as that of chlorine, this mineral oxychloride solution can easily break down carbon bonds and simultaneously lowers organic carbon, meets microbial reduction goals, and complies with the regulatory limits on disinfection byproducts. Disinfection by chlorine can be problematic, as chlorine can react with naturally occurring organic compounds resulting in byproducts that have never existed in nature.
Halogens
On December 16, 1974, the U.S. Congress passed the Safe Drinking Water Act (SDWA), the first set of federally enforceable drinking water regulations that resulted from national concerns to protect drinking water. Chlorination has been the most frequently used form of disinfection for treating drinking water in the U.S., and it has a long history of successful use for inactivation of various microorganisms. When chlorine-based compounds are added to water they react and form hypochlorite ions and hypochlorous acid. If bromine is present, then hypobromous acid is also formed. Along with iodine and fluoride, they are in the “halogen” group of five toxic, non-metal elements with similar chemical characteristics as oxidizing agents. In water, they carry out chemical reactions with a wide variety of compounds and are regarded as effective disinfectants.
Discovery
In 1971, the American scientist Thomas A. Bellar discovered that chloroform was absent in the Ohio River water, which was used for drinking water production. However, chloroform appeared to be present in the drinking water originating from purification plants. This observation proved the formation of chloroform during chlorination. Coincident with the passage of the SDWA, an analytical chemist named J.J. Rook (1974), nearly simultaneously with Bellar and James J. Lichtenberg (1974), formally reported the identification of chloroform and the other trihalomethanes (THMs) as disinfection byproducts formed in chlorinated drinking water resulting from the interaction of chlorine with natural organic matter in water. Disinfection byproducts (DBPs) are chemical, organic, and inorganic substances that can form during a reaction of an oxidant with organic substances known as natural organic matter (NOM) that are present in the water. They essentially arise from natural color and algal metabolic products. The level of organic matter is usually registered as the total or dissolved organic carbon concentration (TOC or DOC), which are the predecessors that ultimately determine the types and concentrations of disinfection byproducts that will eventually be formed.
The discovery of the first DBPs led to research on other chemicals and to their health effects. A modern American scientist, Susan Richardson, later identified greater than 600 water disinfection byproducts in chlorinated tap water, including haloacetic acids (HAAs). Humans are exposed to DBPs through drinking water and oral, dermal, and inhalational contact with chlorinated water.
The four primary trihalomethanes are chloroform, bromodichloromethane, dibromochloromethane, and bromoform. At the center of each is a carbon atom, and it is bound to one hydrogen and three halogens. These four compounds are collectively termed trihalomethanes.
Regulation
THMs and HAAs are currently used as indicator chemicals for all potentially harmful compounds formed by the addition of chlorine to water, even though recent studies have identified several unregulated emerging DBPs that may be more toxic than some of the regulated ones.
The regulation of THMs (and HAAs in the U.S.) was based on their use as ‘surrogates’ for the toxicity associated with chlorinated water. Also, it was known that the control of THMs and HAAs also resulted in a reduction in the concentration of many other DBPs, as well as unknown chlorination DBPs, which may be associated with adverse health effects. However, recent studies suggest that certain emerging DBPs responsible for potential health concerns can be formed when alternative disinfectants to chlorine are used to minimize the formation of the regulated chlorination DBPs. Thus, a growing challenge of concern has been finding ways to cost-effectively control the formation of regulated and emerging DBPs, while meeting disinfection and other operational requirements.
Whatever control measures are adopted, the WHO Guidelines emphasize that the disinfection process must not be compromised and that ‘inadequate disinfection in order not to elevate the DBP level is not acceptable’.
Chemistry
The vast number of chemical reactions can be classified in any number of ways. Under one scheme, they can be categorized either as oxidation-reduction or electron transfer reactions, or non-oxidation-reduction reactions. The term “redox” comes from two concepts involved with electron transfer: reduction and oxidation. These processes are defined as follows: Oxidation is the loss of electrons or an increase in oxidation state by a molecule, atom, or ion. Reduction is the gain of electrons or a decrease in oxidation state by a molecule, atom, or ion.
There is another, different classification scheme for which chlorine can react in three different ways in solutions of organic compounds:
Combination
Combination or substitution reactions involve combining elements to form a chemical compound. Oxidation and reduction occur together: A+B→ABA+B→AB
In a combination reaction, when chlorine reacts by mechanism of addition, chlorine atoms are added to a compound. In a substitution, chlorine atoms are replaced for some other atom that is present in the organic reactant. Both reactions, combination and substitution, produce chlorinated organic compounds and can form DBPs. Disinfection byproducts can also be formed through the reaction of chemical disinfectants other than chlorine. Ozone, for example, can form DBPs when reacting with bromide. The DBP precursor can be either organic or inorganic. In the case of an inorganic precursor, it is most often certain halide ions that transform by oxidation of a complex NOM molecule into a simpler substance and are then reactive with additional chlorine or chloramines acting as a halogen substitution agent. Some of the disinfectants can also oxidize certain halide ions, bromide and iodide to bromine and iodine, which are less effective oxidants but more effective substitution agents than chlorine. In a mixture of chlorine and bromide and/or iodide, mixed chlorine-, bromine- and/or iodine-containing DBP species are formed.
Decomposition
Decomposition reactions are the reverse of combination reactions, meaning they are the oxidation breakdown of a chemical compound into its component elements: AB→A+B.
In a decomposition reaction, when chlorine completes an oxidation reaction, the end products are not chlorinated organic compounds and they account for most of the chlorine demand. The completion of this oxidation reaction does not mean that the result reached the highest oxidation state. It only means that chlorine completed an oxidation reaction and broke down the original compound into two or more simpler forms. In these reactions, chlorination caused a chemical breakdown of the organic compound and no byproducts are formed. That is not to say that the products of the chemical degradation cannot be harmful; it means they are not chlorinated disinfection byproducts.
Displacement
It can be a single substitution when an element in the reactants gets replaced with another element in the products: A+BC→AB+C. A double replacement changes two elements in the reactants, with two in the products: AB+CD→AD+CB.
The Game
When chemical disinfection is applied to water-containing precursors, the formation of DBPs is influenced by different factors:
Precursors: NOM concentration as dissolved organic carbon DOC — the presence of halides affects the formation of halogenated DBPs. When a chlorine-based disinfectant is used, the chlorine residual is an influential factor — the reaction rate is favored by elevated free chlorine residual and precursor concentrations at TOC > 4 mg/L will generate THM exceeding 100 μg/l for a travel time of two to three days in distribution if a free residual is to be maintained at the tap.
pH: THM is also favored by alkaline pH — at pH 9 about 10 to 20 percent more THM will form than at pH 7; on the contrary, HAA formation decreases with increase in pH, but dichloroacetic acid formation is independent of pH.
Temperature: It is favored by increase in temperature. Below 10°C there is no appreciable increase in the formation of DBPs.
Time: An extended contact time increases formation of DBPs.
Alternatively, in the case of chloramine, since it does not react with organic matter to produce DBPs to the same extent as free chlorine, the chlorine dose may be kept low enough to produce only chloramines by making use of ammonia when present in the water, or by using chloramination of raw water. If relatively high levels of DBPs are expected at the treatment works outlet, the degree of removal of organic substances prior to chlorination must be maximized. In some instances, it has been enough to move the pre-chlorination dosing point farther downstream in the treatment process — e.g., after coagulation and clarification have removed a high proportion of DBP precursors. If that is insufficient, advanced treatment processes can be used to prevent DBP formation by the removal of precursors before final chlorination is applied.
The maintenance of residual chlorine in the treated water may cause DBP formation to continue during storage periods and within the distribution system by the reaction with residual precursors. There is some evidence that HAAs undergo some biodegradation in distribution. DBP formation reaction is initially fast, with up to 50 percent formation in the first hour or so, but takes several hours or even days to complete and therefore its concentration at the consumer's tap could be much higher than in the treated water leaving the works. The position can be exacerbated in nutrient-rich waters when ‘booster chlorination’ — i.e., addition of further chlorine at some key point(s) in the distribution system — must be adopted to limit biological aftergrowths within the system. The formation of THMs in the distribution system can be minimized by controlled dosing of ammonia to convert free chlorine residual to combined chlorine residual. However, monochloramine increases the formation of HAA.
Limits to DBP concentrations in drinking water have been set in many countries because of their possible health effects. Emphasis is now placed on avoiding or limiting the pre-chlorination of a raw water containing organic matter in order to minimize the formation of DBPs. If a pre-disinfection stage is necessary, chlorine dioxide or ozone have been the preferred choices so far instead of chlorine. The specific reactions by which many DBPs are produced in chlorinated natural waters are not well understood because the chemical structures of the precursor organic compounds are highly varied and extremely complex.
Health Effects
But one must ask: Why exactly are DBPs being formed to begin with, and why are they detrimental to our health? The answer is very simple. Organic compounds were never supposed to have such a strong presence in the drinking water source. Furthermore, organic compounds and chlorinated compounds were never supposed to meet. Humans added chlorine to the water. It does not happen naturally in nature; it is a manmade act. Chlorine is a good disinfectant and does a good job at killing microorganisms in clean water, but when the water is not clean and other compounds are present, its oxidation potential is not enough to complete oxidation to the highest state and achieve mineralization. People call this “chlorine selectivity” — and, because of misleading marketing campaigns, this has been presented as a quality feature!
Chlorination is capable of oxidizing some of the compounds by chemical breakdown; others it can merely disturb, and is only able to get on board with them (join the enemy by addition?) or do a tradeoff (displacement reaction). When combination or displacement reactions take place, the end product is not a result of complete oxidation — it is an intermediate or byproduct of disinfection. What can result from the interchange of two compounds that were never supposed to come in contact in nature? They will create a new chemical that did not exist in nature before and our body will not recognize it since there is no genetic memory of its presence scripted in our DNA. These new chemicals born to an incomplete oxidation sequence are perceived as invaders to our immune system. The alarm goes off and our bodies feel they are under attack and take proper action to eliminate the stranger, which already started interfering with our metabolic processes in many ways. We have not fully understood the extent of the impact of DBPs to our health. And please keep two things in mind: boiling the water does not solve the problem, and it may in fact exacerbate it by producing more recalcitrant DBPs; and ingesting the water is not the only way that DBPs can get into our body. It is well substantiated that DBPs are absorbed through the skin during bath and showering in enough amounts to affect negatively our health just as if they were ingested and maybe even more. In populations who take hot showers or baths, inhalation and dermal absorption in the shower accounts for more exposure to THMs than drinking water.
The Solution
Advanced oxidation processes (AOPs) for the control of disinfection byproducts
AOPs are globally applied successfully for the removal and degradation of toxic pollutants and to convert recalcitrant contaminants into biodegradable compounds that can then be treated by conventional mechanical, chemical, and biological methods. Their efficacy relies on the generation of highly reactive free radicals, the most important of which is the hydroxyl radical (HO•). The presence of background dissolved organic matter (DOM) is detrimental to the overall performance of AOPs, and not much is known about the molecular-level interaction and reactivity with DOM.
The newest advanced oxidation technology in the market is a mineral oxychloride solution based on Fenton-Haber-Weiss principles of transition mineral catalyzed reactions for the generation of reactive oxygen species. In the formulation, the redox equilibrium is perpetuated by the catalytic activity of mineral-oxygen chelates that provide continued residual protection.
The advanced oxidation process of this new mineral oxychloride solution can simultaneously lower total organic carbon and eliminate 6-log microbial contamination within seconds.
It has the highest hydroxyl radical production among all chemically catalyzed AOPs. At the same time, the hydroxyl radical has the highest oxidation potential among all reactive oxygen species. The reaction speed of OH-radicals is much higher than that of ozone. While ozone has a reaction speed between 1 and 103 L mol-1 s-1 for HO• radicals, the reaction speed lies between 108 and 1010 L mol-1 s-1. Hydroxyl radicals oxidize most organic compounds almost at reaction rates. This means that as soon as HO• encounters organic matter, it will break them down. It is largely non-selective and will react by decomposition with most compounds it will encounter on its path. When HO• is present in sufficient amounts, the AOP will pursue chemical breakdown and degradation into simpler compounds until reaching the highest oxidation state and be completely mineralized in media. The activation of HO• is a very complex process; especially in the case of the mineral oxychloride solution, the generation takes place according to a variety of different reaction mechanisms that involve the production of significant amounts of other ROS with active participation in the overall oxidation proceedings. Residuals are CO2, O2, H2O, mineral salts, and trace nutrients.
In practice, complete mineralization of compounds can be very expensive. In sizable operations, it has not been a popular option due to the high cost of the initial equipment investment and ongoing energy consumption. But this is no longer the case. Since its inception in the market in 2013, the mineral oxychloride solution has made advanced oxidation a viable economic alternative at all flow capacities and scaling operations. With a negligible footprint and same energy consumption as a light bulb, it only requires a liquid chemical dosing system for its implementation. The operation can be fully automated and controlled remotely, as well as easily customized to various target goals. It can be used to enhance oxidation without seeking complete mineralization and in combination with other already established practices. Overall, the superior oxidation will improve the effectivity of downstream purification processes including bioreactors. Some operations control the chemical dosing to free certain compounds of unwanted effects by partial oxidation — like color, taste, and scent — and others administer for pre-biodegradation of various organic compounds. The mineral oxychloride solution used as pre-oxidant in a water treatment plant will lower the dissolved organic matter; and. with monitoring of oxidation-reduction potential (ORP), you can have an affordable solution to control the formation of DBPs in the final disinfection phase and avoid violations while gaining a superior quality of the water.
The mineral oxychloride solution is sold with the trademark Biohydrox™ by Envirocleen LLC (www.envirocleen.com).
About the Author
Emma Flanagan is a water consulting professional specializing in applications of homogeneous catalytic generation of reactive oxygen species with transition minerals. She holds an MS degree in sanitary engineering from IHE Delft Institute for Water Education in the Netherlands, the largest international graduate water education facility in the world. Emma stays busy doing research, educating, and providing consulting services.