Doing More With Less: Densified Activated Sludge (DAS) Systems For Water Resource Recovery Facilities
Jointly authored by members of Hazen and Sawyer (Gaya Ram Mohan, Wendell Khunjar, Ron Latimer, Yewei Sun, Alonso Griborio, Alyssa Mayer, Will Martin, Ben Levin, Joe Rohrbacher), Portland Water District (Scott Firmin), Gwinnett County Department of Water Resources (David Jones), and Metro Water Recovery (Rudy Maltos, Blair Wisdom, Dan Freedman, Liam Cavanaugh)
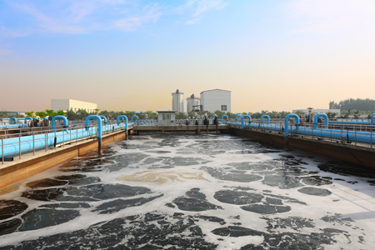
As wastewater treatment plants — or water resource recovery facilities (WRRFs), more preferably termed — continue to strive for efficiency, DAS has emerged as a space-saving intensification solution.
There are approximately 14,000 WRRFs in the U.S., 5,000 of which possess permits that include some form of nutrient limit on their discharge (EPA, 2012). Recognizing that WRRFs account for 10% to 15% of the overall nutrient load to surface waterways in the U.S., utilities are increasingly challenged with meeting new or lower nutrient effluent limits. A key challenge and opportunity for a growing number of utilities is to find financially responsible and effective nutrient treatment.
Biological treatment via activated sludge has been a backbone of WRRFs, exploiting the selection of various functional groups of bacteria that have the ability to remove contaminants like organic carbon, nutrients (nitrogen and phosphorus), and pathogens. A core principle behind the success of activated sludge is effective solids/water separation to retain the microorganisms and produce an effluent free of soluble and particulate contaminants. Solids/water separation has historically been achieved by gravity via clarifiers/settlers and filters with more recent use of membrane separation.
For activated sludge systems that rely on gravimetric separation of solids and water, the rate at which sludge settles and compresses is a critical factor that influences how WRRF infrastructure is sized and operated. Densification of activated sludge has emerged as a strategy for WRRFs seeking to improve the rate-limiting step of gravimetric settling to unlock treatment capacity and achieve process intensification. Densification is the process of growing and retaining particles with improved settling characteristics. Densification improves sludge-settling characteristics over conventional activated sludge by allowing operation at high mixed liquor suspended solids (MLSS) concentrations (e.g., 4,000 to 8,000 mg/L) while still employing gravimetric settling (e.g., secondary clarifiers or sequential batch reactors). Process intensification via densification allows utilities to optimize the use of existing facilities and reduce the need for additional infrastructure (e.g., tanks, equipment) while also potentially reducing energy and chemical consumption.
For these reasons, densified activated sludge (DAS) systems have received widespread interest within the water sector. For example, the aerobic granular sludge (AGS) process that was developed via a public/private partnership in the Netherlands and branded as Nereda and AquaNereda has become widely applied across the globe, with over 90 full-scale plants as of 2022 (Pronk et al., 2015). The current AGS technology utilizes sequencing batch reactors (SBRs), where plug-flow feeding and washout of slow-settling particles (i.e., tall reactors with short settling time) are applied to produce exopolymeric substances (EPS).
DAS can also be achieved in non-SBR configurations. Recent work has shown successful densification at lab-, pilot-, and full-scale in a variety of shallow, completely mixed, continuous-flow bioreactors and clarifier configurations (Avila et al., 2021; Daigger et al., 2018; Sturm, 2020; Wei et al., 2021b); (Li et al., 2015; Sun et al., 2019; Xu et al., 2020; Zou et al., 2018) (Avila et al., 2021).
Figure 1. Continuum of activated sludge-settling characteristics
What Is Densified Activated Sludge?
Activated sludge systems can experience a wide range of settling conditions, ranging from filamentous bulking to flocculant to partially densified to fully granular. DAS terminology represents a continuum of settling conditions that generally are characterized as having rapid settling velocities and low variability in longterm settling properties and whose composition comprises a combination of flocs and granules (Figure 1). AGS systems like Nereda/AquaNereda represent a highly intensified version of DAS whereby granules (particles > 212 µm) comprise greater than 70% of the sludge matrix.
What Factors Contribute To Full-Scale Densification?
Several factors have been identified as being critical to achieve densification:
Control of selector zone food to microorganism (F/M) ratio and feast to famine regimes: Biological selection forms the basis behind DAS. Providing appropriate-sized zones (kinetic selection) allows selection for organisms that rapidly uptake readily biodegradable substrate under anaerobic, anoxic, or aerobic conditions (metabolic selection) to produce alginate like extracellular polysaccharides (EPS) (Lin et al., 2010) and internal carbon storage products. Famine conditions are then required to allow organisms to consume stored products, which facilitates structured growth into dense particles.
Control of shear conditions in properly loaded systems: Shear forces have also been shown to influence the production of EPS in biological systems as well as the abrasion rate of particles. The combination of biological and shear force selective pressures can therefore yield compact and dense particles that increase rate of settling in activated sludge.
Selective wasting of biomass to retain dense particles: Physical selection acts to preferentially retain fast-settling particles while wasting the slow-settling fraction and surface-frothtype organisms. This approach can result in the decoupling of solids retention times between the flocculant and granular phases. Several strategies have been employed in practice to achieve selective wasting, namely surface-wasting, hydrocyclones, lamella plates, upflow settlers, and mechanical sieves.
Laying the Ingredients for Densification at the East End Wastewater Treatment Facility
Portland Water District’s (PWD) East End Wastewater Treatment Facility (20.8 MGD rated capacity), located in the Presumpscot watershed of Casco Bay, previously experienced sludge settleability issues caused by inadequate dissolved oxygen levels and excessive filamentous growth in the biological treatment process. PWD conducted a study to determine upgrades to improve sludge settleability and process reliability as related to nitrification.
PWD installed fine-bubble diffusers to improve air delivery to the biological treatment process and added kinetic and metabolic selector zones to reduce sludge bulking. Since completion of the aeration upgrade, average sludge value index (SVI) values reduced from 250 mL/g to around 120 mL/g.1 Ammonia levels of less than 1 mg/L have been consistently observed since the improvement in settleability have allowed PWD to more reliably maintain solids inventory necessary to achieve nitrification. This project demonstrates the value of utilizing biological selection as a means for facilitating the early stages of densification — i.e., the development of small and compact flocs.
Leveraging Densification for Treatment Capacity at the Crooked Creek Water Reclamation Facility
Crooked Creek Water Reclamation Facility (CCWRF), owned and operated by Gwinnett County Department of Water Resources, is designed to treat 16 MGD using an anaerobic-anoxic-aerobic (A2O) configuration. The facility does not practice anaerobic digestion and has consistently achieved measurements of effluent ammonia (NH3-N) and total phosphorus (TP) less than 1 mg/L and 0.3 mg/L, respectively.
The older oxidation-ditch-type plant was upgraded in 2021 to an A2O process with a design emphasis on promoting DAS; and since start-up of the new bioreactors, the SVI at the 50th and 95th percentiles have remained low at 62 and 78 mL/g, respectively.2 Through the use of DAS, it is anticipated that the CCWRF can be re-rated from 16 to 25 MGD without the need for new bioreactor basins. Results from this plant indicate that it is possible to achieve stable densification in continuous flow applications using kinetic and metabolic selection only.
Positioning for the Future at the Robert W. Hite Treatment Facility
Metro Water Recovery’s (Metro) Robert W. Hite Treatment Facility (RWHTF) is a 220 MGD facility that currently achieves effluent total inorganic nitrogen (TIN) < 10 mg/L and total phosphorus (TP) < 1 mg/L. Metro commissioned a full-scale, continuous-flow DAS demonstration train to understand the role that DAS can play in addressing anticipated reduction in effluent nutrient limits over the next 20 years.
Results have confirmed that DAS improved settling characteristics and would allow Metro to load clarifiers at surface overflow rates (SORs) > 700 gallons per day per square foot (gpd/ sf) and solids loading rates (SLRs) > 60 pounds per day per square foot (ppd/sf), nearly twice current loading limits.3 This could allow Metro to defer construction of new aeration tanks and clarifiers, treating the wastewater to a higher quality and within the existing footprint. Results from Metro demonstrate that DAS can best be achieved using a combination of metabolic, kinetic, and physical selection.
Densify To Intensify
As utilities consider the future of treatment at WRRFs, DAS implementation should be considered to:
- Improve settleability with a view to increasing capacity of existing infrastructure, while also improving nutrient removal capabilities.
- Reduce sizing of new WRRFs, given the smaller, more efficient nature of biological nutrient removal (BNR), secondary clarifier equipment, and facilities.
- Position utilities to synchronize nitrification/densification/phosphorus uptake processes, which will reduce energy costs, carbon outputs, and greenhouse gas (GHG) emissions.
References
- Levin et al., 2019. Sludge Settleability Improvements at the East End WWTF. New England Water Environment Association Annual Conference.
- Latimer et al., 2022. Continuous Flow Densification of Activated Sludge at Crooked Creek Water Reclamation Facility. WEFTEC 2022.
- Mayer et al., 2022. Leveraging Full-Scale Continuous Flow Densification of Activated Sludge at Robert W. Hite Treatment Facility for Meeting Long Term Treatment and Capacity Needs. WEFTEC 2022.
Pronk, M., de Kreuk, M.K., de Bruin, B., Kamminga, P., Kleerebezem, R. and van Loosdrecht, M.C.M. 2015. Full scale performance of the aerobic granular sludge process for sewage treatment. Water Res. 84, 207-217.
Avila, I., Freedman, D., Johnston, J., Wisdom, B. and McQuarrie, J. 2021. Inducing granulation within a full-scale activated sludge system to improve settling. Water Science and Technology.
Daigger, G.T., Redmond, E. and Downing, L. 2018. Enhanced settling in activated sludge: design and operation considerations. Water Science and Technology 78(2), 247-258.
Sturm, B. 2020. Balancing Flocs and Granules for Activated Sludge Process Intensification in Plug Flow Configurations. Water Environment Research Foundation Project U1R14.
Wei, S.P., Stensel, H.D., Ziels, R.M., Herrera, S., Lee, and Winkler, 2021b. Partitioning of nutrient removal contribution between granules and flocs in a hybrid granular activated sludge system. Water Research 203, 117514.
Li, J., Cai, A., Ding, L., Sellamuthu, B. and Perreault, J. 2015. Aerobic sludge granulation in a Reverse Flow Baffled Reactor (RFBR) operated in continuous-flow mode for wastewater treatment. Separation and Purification Technology 149, 437-444.
Sun, Y., Angelotti, B. and Wang, 2019. Continuous-flow aerobic granulation in plug-flow bioreactors fed with real domestic wastewater. Science of the Total Environment 688, 762-770.
Xu, D., Li, J., Liu, J. and Ma, T. 2020. Rapid aerobic sludge granulation in an integrated oxidation ditch with two-zone clarifiers. Water research 175, 115704.
Zou, J., Tao, Y., Li, J., Wu, S. and Ni, Y. 2018. Cultivating aerobic granular sludge in a developed continuous-flow reactor with two-zone sedimentation tank treating real and low-strength wastewater. Bioresource technology 247, 776-783.
Jenkins, D., Richard, M.G. and Daigger, G.T. (2003) Manual on the causes and control of activated sludge bulking, foaming, and other solids separation problems, Crc Press.
Lin, Y., de Kreuk, M., Van Loosdrecht, M. and Adin, A. 2010. Characterization of alginate-like exopolysaccharides isolated from aerobic granular sludge in pilotplant. Water research 44(11), 3355-3364.
Chudoba, J., Blaha, J. and MadÄ›ra, V. 1974. Control of activated sludge filamentous bulking—III. Effect of sludge loading. Water Research 8(4), 231-237.
Chudoba, J., Ottova, V. and Madera, V. 1973. Control of activated sludge filamentous bulking—I. Effect of the hydraulic regime or degree of mixing in an aeration tank. Water Research 7(8), 1163-1182.