Advanced Aeration In Hydraulic Fractured Water Impoundments
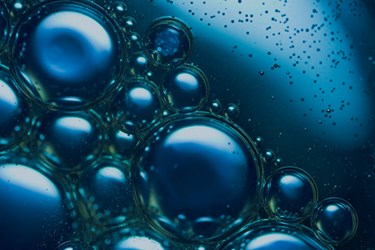
With so much attention paid to the fate of frack water — how it’s handled and cleaned — it’s time to consider the effectiveness of prevailing treatment methods.
By Tom Daugherty, head of corporate development, Arcadian Technologies, LLC.
Most hydraulic fractured water (flowback) impoundments use air in some sort of treatment scheme. Many observers maintain that it is intuitive to inject a lifeless body of water with air. Evaporation of water is the primary management objective of most flowback impoundments, and site management endeavors to increase evaporation. Various aeration schemes systematically churn water because it is widely believed that doing so promotes evaporation. Deployed irrigation systems are generally a pumped waterfall back into the impoundment. This article will explore the quantifiable benefits of aeration.
Baseline: The Cycle Of Water
The water we have on Earth is all we have. New water is not created as years pass by. The hydrologic cycle well explains the circuitous route that water takes. Water that is here now is consumed, transpired, or evaporated, then journeys away only to come back again as precipitation or groundwater to start the cycle again. In municipal water treatment, water is simply a transport mechanism. It was clean to start with, prior to washing clothes or flushing the toilet. Reclamation facilities exist to return the water to its natural state and discharge it to a local water body. And the water cycle goes on.
Dissolved Oxygen
By merely looking at water you cannot tell if it has oxygen in it other than the hydrogen and oxygen molecules. But oxygen is not the molecule that improves water quality and allows aquatic biota to survive. Dissolved oxygen (DO) must also be present in ample concentration to support robust water health and is widely understood as a powerful oxidant. Even a stagnant pond can be brought back to life in short order by effectively infusing DO into the water. In nature, the cascading of a mountain stream over rocks enriches the DO level, ensuring that trophy species of fish thrive. Healthy DO levels promote beneficial biologic activity from naturally occurring microbes. Increasing dissolved oxygen in flowback impoundments initiates a plethora of water quality improvements. Let’s examine them one-by-one.
Evaporation
Many centralized treatment ponds are also known as evaporative ponds or pits. Two key components to evaporation in a water impoundment are heat (sunshine) and surface air movement (wind). Other factors include altitude, vapor pressure at the water surface, and vapor pressure of the surrounding air.1 One can discover a myriad of evaporation formulas based on geography, latitude, climate, and the like, but the aforementioned factors are in play whether you are in the Permian Basin, Marcellus Shale, or elsewhere. During interviews with three evaporation pit operators, it was discovered that none deployed any scientific methodology to determine evaporation rate, but all were convinced that their aeration methods helped.
Advanced Aeration Improves Evaporation
There are multiple phenomena occurring when microbubbles and coarse bubbles are introduced in a subsurface fashion in a water impoundment. First, the rising bubbles cause surface disruption and may add momentum to the air, increasing the rate at which the humid air is removed from the surface, a critical aid to evaporation.2 Second, when air is introduced into water, bubbles are formed, and diffusing vapor in the water migrates to the interior of the bubble.3 The resultant migrant vapor in the bubble reaches 100 percent relative humidity and is released during the breakup process at the surface.4 The combination of coarse and fine bubbles delivered from the water pit floor, effectively destratifying the entire water column, is considered advanced aeration.
As mentioned earlier, a casual observation of existing fractured water impoundments notes some sort of surface aeration, weeping systems, periodic air jamming, or other air sparging. By field practice, the operators realize the advantages of utilizing air in water management.
Score Card For Aeration Efficiency
In 1984 the American Society of Civil Engineers (ASCE) adopted aeration efficiency standards. The Standard Oxygen Transfer Efficiency (SOTE) test was developed to put all vendors/ methods on an even playing field. The key to SOTE is evaluating the efficiency of introducing oxygen into a body of water. The trial is recorded at sea level in clean water at 20 degrees C. Bubble size is the single biggest factor to determine SOTE. Fine bubbles (< 1/8”) tend to rise more slowly, providing better oxygen transfer efficiency. Coarse bubbles (>1/4” and often 1” to 2”) are more effective at mixing. Other factors in analyzing an aeration system are flow rate, depth, temperature, elevation, layout, alpha and beta factors, and maintenance. Chart 1 shows the Standard Aeration Efficiency (SAE) for various aeration types at different water depths. Keep in mind the higher SAE rating is the most desired, as it takes into account implied utility costs. The measurement is pounds of oxygen per horsepower per hour expressed as lb O2/hp-hr.
Chart 1: Nature’s Oxidant And Water Quality Improvements
When advanced aeration is introduced to flowback water, many water quality parameters begin improving in correlation to residence time or exposure. Microbubbles can effectively introduce DO into the target water body. Assuming anaerobic conditions, a transformation from anaerobic (DO < 1.0 mg/L) to aerobic (DO > 1.0 mg/L) water occurs. Sulfate-reducing bacteria (SRB) are susceptible to the oxidation potential of DO.5 Initiating the oxidation of SRB inhibits the formation of hydrogen sulfide, a colorless gas that is corrosive, poisonous, flammable, and explosive. Effective infusion of DO also begins the oxidation of hydrogen sulfide, which helps mitigate multiple issues, including odor.6 With increased DO, the pH level in the water body begins to move toward stabilization. This is valuable when it is understood that a target pH value between 7.0 and 8.0 is optimum for maximum hydrogen sulfide removal.7 The water body also begins stripping CO2, light hydrocarbon gases, and VOCs due to redox, which is a reflection of its oxidative and reductive capacity.
Dissolved oxygen transforms metals such as iron and manganese to their oxidized state. This allows them to be filtered out or settled to the bottom of the pit. Guar residues and other organics also begin oxidizing, which fosters increased light transmissivity or clarity in the water. These documented water quality improvements offer contaminant load reduction for follow-on treatment trains, such as electro-coagulation, reverse osmosis (RO), and the like.
The surface disruption caused by aeration also reduces surface matting and encrustable formations. This benefit is twofold, as it allows more access to the water body by UV rays and provides a naturally corralled surface area for additional skim harvesting of hydrocarbons.
Anaerobic water pits cause certain treatment chemicals to be oxidized or partially consumed. Often they suffer “serendipitous uptake” by nontarget contaminants. This causes them to be less effective than expected, leading to costly overdosing. Increasing DO with advanced aeration will optimize subsequent chemical use and increase operator control and understanding of water pit behavior.
Taking control of fractured water impoundments is a critical first step in subsequent treatment, recycling, evaporation, or other intended reclamation of the water. Cost control and process improvement are to be gained by addressing the water chemistry early on in the process, promoting improvement each step of the way. A cursory observation of the literature coupled with the data presented in chart 1 suggests that using aeration deploying coarse and fine bubbles would offer the water quality benefits outlined herein. Notwithstanding nuances from site to site, advanced aeration merits serious consideration in the continuum of fractured water management.
About The Author
Tom Daugherty has been involved in the environmental industry for the past 12 years, and is currently head of corporate development at Arcadian Technologies, LLC. Daugherty was formerly president of Blue Water Technologies. He holds an MBA in sustainability from Marylhurst University. He can be reached at tomd@arcadiantech.com.
References
1. Potts, D., (1988). Estimation of Evaporation from shallow ponds & impoundments in Montana. Misc pub #48. Montana Forest and Conservation Experiment Station. School of Forestry, University of Montana
2. Brutsaert, W., (1982). Evaporation into the atmosphere. Dordrect, Reidel. p. 299
3. Burkard, M.E. & Van Liew, H.D., (1994). Simulation of exchanges of multiple gases in bubbles in the body. Resp. Physiol., 95 (2). pp. 131-145
4. Helfer, F., Lemckert, C., and Zhang, H., (2012). Influence of bubble plumes on evaporation from non-stratified waters. Journal of Hydrology. Volumes 438–439, 17 May 2012, pp. 84–96
5. Characklis, L., Lee, W., (1994). Review article on the influence of dissolved oxygen on sulfate-reducing bacteria-related corrosion. Mycotoxins, Wood Decay, Plant Stress, Biocorrosion, and General Biodeterioration, Biodeterioration Research Volume 4, 1994, pp. 519-526
6. Sharma, K., Yuan, K., (2010) Kinetics of chemical sulfide oxidation under high dissolved oxygen levels, 6th International Conference on Sewer Processes and Networks. Gold Coast, Australia. Nov. 7-9, 2010
7. Chen, K., Morris, J., (1972). Kinetics of aqueous sulfide by O2. Environ. Sci. Technol., 1972, 6 (6), pp. 529–537