Shall We Compromise The Health Effect Of Disinfection Byproducts To Control Waterborne Diseases?
By Emma Flanagan
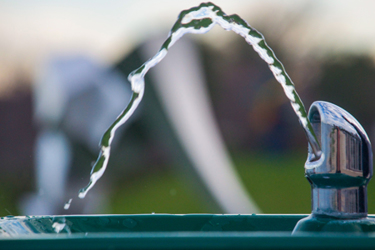
Disinfection has come a long way — enough so to consider alternative options that can help water suppliers avoid harmful byproducts.
While chlorine-based disinfectants are the most commonly used, not a single one is always effective against all pathogens, biofilms, and oxidizable compounds. All oxidizing disinfectants generate disinfection byproducts (DBPs), which pose potential health risks of their own. Currently, most drinking water regulations aggressively address DBP problems in public water distribution systems, with the most concern placed on trihalomethanes (THMs), haloacetic acids (HAAs), bromate, and chlorite. The World Health Organization, via WHO Guidelines for Drinking Water Quality, recommends that water disinfection should never be compromised by attempting to control DBPs. The risks of human illness and death from pathogens in drinking water are much greater than those from exposure to disinfectants and disinfection byproducts. Nevertheless, if DBP levels exceed regulatory limits, strategies should focus on eliminating organic impurities that foster their formation without compromising disinfection.
The Chlorine Dilemma Continues
Long gone are the times when good taste and smell were the guarantees of safe drinking water. In 1854, the British scientist John Snow discovered that cholera was spread through contaminated water. He applied chlorine to purify the water, which paved the way for the future of water treatment … happily ever after until 1971, when the American scientist Thomas A. Bellar discovered that chlorination in a drinking water treatment plant with a source from the Ohio River was creating disinfection byproducts in the form of chloroform. When in 1972 the Clean Water Act was introduced in the U.S., the discovery of more DBPs followed soon after.
When it comes down to water disinfection, there has been an interesting trend over the past several decades. More and more water treatment facilities are switching from chlorination to other means of disinfection. Based on efficiency, UV, ozone, and chlorine dioxide are the most efficient overall as broad disinfectants. Each has unique advantages and disadvantages in cost, efficacy-stability, ease of application, and the nature of DBPs. Interestingly, all current chemical disinfection methods are associated with byproducts, but chlorinated DBPs are the most well studied.
The Case Of UV Radiation
When UV light-based reactors were discovered, the costs for installing and maintaining them were high. Over the years, development in the UV sector has decreased the costs significantly, and UV-radiation optimization has made this a viable method for water disinfection. After many years of research, it is recognized as one of the most effective alternative water disinfection processes available. UV radiation does not kill the micro-organisms directly, but it damages their DNA and RNA. By doing this, the micro-organism can still function; however, it cannot use its DNA/RNA to reproduce, causing the organisms to become extinct. UV disinfection is effective at inactivating most micro-organisms that are present in the water. What remains is often removed by adding oxidants.
One of the most important advantages is that it does not produce any known toxic byproducts. Another advantage of UV disinfection is that the effectiveness is relatively insensitive to temperature and pH differences. Also, UV installations require little supervision or maintenance. Its benefits include improved safety, minimum service time, low operation and maintenance costs, and no chemical taste or smell to the disinfected water. Two key disadvantages have to be taken into account when considering UV radiation disinfection. Probably the most crucial disadvantage is that UV light can be scattered and therefore be ineffective in neutralizing micro-organisms. Suspended solids, turbidity, color, or soluble organic matter can cause this. Turbidity also limits the penetration depth of the radiation in the water. To limit these problems, precautions have to be taken, such as filtering of the water and reducing turbidity. A second substantial disadvantage is the absence of any residual disinfectant in the water, which means that unless the water is transported through a micro-organism-free environment, there is a chance of recontamination. Another disadvantage of UV disinfection is the complicated process of measuring its effectiveness.
The Case Of Ozone
Ozone is an excellent broad-spectrum and fast disinfectant, as well as an excellent oxidizer. It is an unstable compound that rapidly decomposes into much more stable oxygen. For the ozonation of drinking water, ozone must be produced onsite. Ozone is generated by discharging a high-voltage current in dry air or pure oxygen (much as in nature, where a distinct ozone smell can be discerned after a thunderstorm). This method yields a maximum of 3% ozone for air, while yields of 12% are achievable with pure oxygen. The ozone is then brought into contact with the water that has to be treated. It is used as a standalone in some cases. It can also be combined with hydrogen peroxide (H2O2), UV light, or active chlorine to perform an advanced oxidation process (AOP). This AOP produces highly reactive hydroxyl radicals (OH•). These reactive species are the most potent oxidants that can be applied to water. They are used to address contaminants with high chemical oxygen demand (COD) or non-biodegradable molecules. They also improve the overall quality of the water. Ozone can be used to complement chlorine disinfection of drinking water, and utilities can reduce the use of chlorine if there’s a concern about dangerous byproduct formation.
Ozone disinfection equipment is more complicated than chlorination systems. It is very costly and has significant energy demand. To ensure proper disinfection and account for gas-solubility ratio, ozone dosage is higher than what is required in the water. About 85% of the energy input to a corona discharge system is wasted in the form of heat. This method is exceptionally energy-intensive, meaning that ozone generation is typically more expensive than the alternatives. Naturally, some very irritating and probably toxic ozone remains in the off-gas after contacting. To release the off-gas safely into the atmosphere, it must be run through an ozone destruction unit. Other disadvantages are that when ozone reacts with organic compounds, it creates a variety of byproducts. If the water contains bromide ions, ozone treatment can form brominated compounds like the bromate ion, a possible human carcinogen. Finally, ozone is unlike chlorine in that there is no residual or remaining disinfectant once the process is over; any ozone that doesn’t react with contaminants breaks down completely. This makes it more difficult for plant operators to keep tabs on how well disinfection is working since there is no residual ozone in the water that they can monitor.
The Case Of Chlorine Dioxide
Chlorine dioxide is another break-off from chlorine that can be used to treat and purify water. More systems worldwide have been turning to chlorine dioxide to treat drinking water to improve taste, odor, and color; remove iron and manganese; and inactivate chlorine-resistant micro-organisms with a limited formation of DBPs.
Chlorine dioxide is a synthetic, green-yellowish gas discovered in England in 1814. It is a small, volatile, and strong molecule with the formula ClO2 and a chlorine-like, irritating odor. However, it is different from chlorine Cl2, both in its chemical structure and in its behavior. It dissociates into chlorine gas (Cl2), oxygen gas (O2), and heat. Diluted in water, chlorine dioxide is a free radical, and when it is photo-oxidized by sunlight, it falls apart. One of the qualities of chlorine dioxide is its high water solubility, especially in cold water. It is approximately 10 times more soluble in water than chlorine. It does not hydrolyze when it enters water; it remains a dissolved gas in solution.
In water treatment, chlorine dioxide is an effective biocide that is strong enough to attack the disulfide bonds found in the membranes of bacteria and other biological materials that play a vital role in micro-organisms, and they are not able to build resistance. It is also very good at attacking biofilms. As an oxidizer compared with other oxidizing biocides, chlorine dioxide has a significantly lower oxidation strength — this means that it reacts with fewer compounds, such as organic compounds and ammonia, which is characterized as selective oxidation.
Research indicates that pure ClO2 used in water with organic content creates lower amounts of DBPs. It does not form THMs but may generate small concentrations of dihalogenated HAAs. A growing body of literature shows that ClO2 preoxidation can help reduce chlorine-related DBPs when chlorine is subsequently applied for primary or secondary disinfection. While test data can look great during lab studies, onsite generation of ClO2 often involves the production of free chlorine, unreacted reagents, or side-reaction products; thus, the disinfectant is a mixture, and the ratios of ClO2 to chlorine significantly affect the chlorine DBPs formed, depending on the properties of the water being treated. Even when the generation of chlorine during onsite production of ClO2 could be negligible, the production of chlorine dioxide, its decomposition, and its reaction with natural organic matter (NOM) still lead to the formation of byproducts of its own, like chlorite and chlorate. Chlorite (ClO2-) is a compound of health concern along with chlorate (ClO3-), which can be harmful in high concentration but is not yet regulated.
Like ozone, chlorine dioxide must be produced onsite, implementation is expensive, and it takes more technical skills to use. Operation requires daily monitoring of chlorite and chlorine dioxide.
The Case Of Advanced Oxidation
Degradation and mineralization of NOM is the best way of controlling the production of DBPs. The highest oxidation state reached by a chemical pollutant will be determined by the oxidation potential of the oxidizing agents. Advanced oxidation is a process that applies enough hydroxyl radicals to achieve significant change at the molecular level. The hydroxyl radical is a reactive oxygen species with the highest oxidation potential among all oxidizing agents used in water treatment. It is mainly non-selective and reacts by decomposition with most compounds it encounters on its path. In 2013, the International Sanitary Foundation certified NSF/ANSI 60, the first advanced oxidation reagent for the treatment of drinking water for human use. This reagent is a multimineral oxychloride liquid formula that does not need to be generated onsite, has low safety risk, and is cheaper and simpler to operate than other chlorine alternatives.
The mineral oxychloride*, MxOyCLz, mimics the oxidation processes in nature. Although it is considered a chlorinated compound because it can produce OCl- and provide a measurable chlorine residual, its active agents are reactive oxygen species in the form of radicals and ionized oxygen with very high oxidation potential. They can complete chemical reactions extremely fast and transform all oxidizable substances into their highest oxidative state. When the mineral oxychloride reacts with water, the residual hypochlorite ion is basically on standby without the opportunity to attack micro-organisms or degrade compounds, and its oxidative potential, since it is not being spent, becomes a backup energy source for residual protection.
The mineral oxychloride is 100 percent soluble in water at all temperatures and will not break down in gas form. It has low sensitivity to pH, and its reactivity increases with the temperature of the water. Rather than being photo-oxidized by sunlight, like chlorinated compounds, it is catalyzed by the sun, and its exposure increases reactivity. The main oxidizing oxygen species that it produces are hydroxyl radicals, oxygen ions, and hydroxyl ions. It also generates large quantities of perhydroxyl and superoxide radicals.
In drinking water treatment, the mineral oxychloride has superior effectiveness to ozonation at a fraction of the cost and without external energy consumption. For this reason, it is sometimes called “liquid ozone.” When comparing to ozonation, however, it is important to note how they differ in the utilization of energy and the formation of DBPs. Ozone generation requires external energy, and in water treatment the process is not very energy efficient, so more energy than what is consumed by the formation of ozone molecules must be applied. The excess energy in the water will excite bromide ions when present and produce bromate. On the other hand, the mineral oxychloride is catalyzed by the internal vibrational energy of transition minerals, which in turn activates the reactive oxygen species, a very energy efficient process whereby all the energy is created and used at the atomic level. There is no excess energy transferring to the water. The mineral oxychloride, even if applied in excess, does not react with bromide ions and does not create bromate.
There are other important conveniences of using mineral oxychloride. It has a minimum shelf life of six months in normal storage conditions, a negligible capital cost (only needs automatic liquid dosing equipment), and a smaller amount will be required to treat the same volume of water since it is a more powerful oxidant than all chlorinated products — comparable to the refractory pollutant degradation that can be obtained with catalyzed hydrogen peroxide or peroxone, without generating any byproducts and while providing robust residual protection. After all, advanced oxidation is, by definition, an oxidation process that has been taken to the next level!
*The mineral oxychloride solution is sold with the trademark Biohydrox ™ by Envirocleen LLC (www.envirocleen.com).
About The Author
Emma Flanagan is a water consulting professional specializing in applications of homogeneous catalytic generation of reactive oxygen species with transition minerals. She holds an M.S. degree in sanitary engineering from IHE Delft Institute for Water Education in the Netherlands, the largest international graduate water education facility in the world. Flanagan stays busy doing research, educating, and providing consulting services.